The effect of strain rate on the determination of mechanical properties of steel bar for reinforced concrete
DOI:
https://doi.org/10.60136/bas.v8.2019.268Keywords:
Strain rate, Strain rate sensitivity, Mechanical propertyAbstract
In tensile test, most of testing standards specify applicable strain rates because of that the materials will yield at different strength levels and are slightly sensitive to the strain rates for the tensile strength. The materials tested in according to the testing standards should present equivalent strength values without considering which test laboratory performs the tensile test. The presented material strength may depend upon the used strain rates. Therefore, the question comes up whether or not different strain rates have an effect on the mechanical properties of the tested materials.
Referring to the testing standard of steel bar for reinforced concrete, it is required to know definite minimum values of the mechanical properties of steel bar for reinforced concrete, such as yield strength, tensile strength, elongation, reduction of area, and elastic modulus. Therefore, testing laboratories have to test for gaining reliable mechanical property information, which will lead to the exact utilization. This can avoid severe damage, loss of life, and high expense in maintenance.
This research study aimed to study the effect of different strain rates (0.000185 s-1, 0.000555 s-1, 0.001296 s-1, 0.002222 s-1, 0.011111 s-1, and 0.02963 s-1) on the mechanical property values (such as yield strength, tensile strength, elongation, and reduction of area) of steel bar for reinforced concrete by considering the strain rate sensitivity (). The experimental results showed that different strain rates had an impact on the mechanical property values. When the strain rate was increased, the tensile strength tended to decrease (around 13 MPa) and the yield strength tended to increase (around 19 MPa), according to the strain rate sensitivity for the tensile strength m=-0.0024 and the strain rate sensitivity for the yield strength m=0.0045, respectively. The increasing of the strain rate had more effect on the yield strength than the tensile strength. In addition, the uncertainties of the yield strength (about ±30 MPa และ ±47 MPa, respectively) and tensile strength (about ±60 MPa และ ±82 MPa, respectively) were higher at the minimum strain rate and the maximum strain rate. Moreover, when the strain rate was increased, the elongation tended to decrease (approximately 3%) and the reduction of area tended to slightly increase (approximately 1%), according to the strain rate sensitivity for the elongation m=-0.0341 and the strain rate sensitivity for the reduction of area m=-0.0032, respectively. When the strain rate was increased, the elongation significantly decreased. In contrast, the reduction of area slightly increased or barely changed. In addition, the uncertainties of the elongation (approximately ±0.2%) and reduction of area (approximately ±0.3%) were risen very slightly, when the strain rate was increased.
References
HUH, J., H. HUH and C. S. LEE. Effect of strain rate on plastic anisotropy of advanced high strength steel sheets. International Journal of Plasticity. May, 2013, 44, 23-46.
LI, X., J. CHEN, L. YE, W. DING and P. SONG, Influence of strain rate on tensile characteristics of SUS304 metastable austenitic stainless steel. ACTA Metallurgica Sinica. 2013, 26(6), 657-662.
ANDERSON, D., S. WINKLER, A. BARDELCIK and M. J. WORSWICK. Influence of triaxiality and strain rate on the failure behaviour of a dual-phase DP780 steel. Materials & Design. August, 2014, 60, 198-207.
BLAZ, L. and E. EVANGELISTA. Strain rate sensitivity of hot deformed Al and AlMgSi alloy. Materials Science and Engineering: A. 1996, 207(2), 95-201.
HADIANFARD, M. J., R. SMERD, S. WINKLER and M.WORSWICK. Effects of strain rate on mechanical properties and failure mechanism. Materials Science and Engineering: A. 2008, 492(1-2), 283-292.
LUO, J., M. Li, W. Yu and H. Li. The variation of strain rate sensitivity exponent and strain hardening exponent in isothermal compression of Ti-6Al-4V alloy. Materials & Design. 2010, 31(2), 741-748.
LICHTENFELD, J. A., M. C. MATAYA and C. J. VAN TYNE. Effect of strain rate on stress-strin behavior of alloy 309 and 304L austenitic stainless stainless steel. Metallurgical and Materials Transactions: A. 2006, 37(1), 147-161.
ZHOU, F., J. N. WANG and J. S. LIAN. An investigation of the plastic failure of spheroidized steels. Materials Science and Engineering: A. 2002, 332(1-2), 117-122.
FAN, G. J., L. F. FU, H. CHOO, P. K. LIAW and N. D. BROWNING. Uniaxial tensile plastic deformation and grain growth of bulk nanocrystalline alloys. Acta Materialia. 2006, 54(18), 4781-4792.
NILSSON, K. F and V. VOKAL. Analysis of ductile cast iron tensile tests to relate ductility variation to casting defects and material microstructure, Materials Science and Engineering: A. 2009, 502(1-2), 54-63.
BACHA, A., D. DANIEL and H. KLOCKER. On the determination of true stress triaxiality in sheet metal. Journal of Materials Processing Technology. 2007, 184(1-3), 272-287.
PUTATUNDA, S. K. Fracture toughness of a high carbon and high silicon steel. Materials Science and Engineering: A. 2001, 297(1–2), 31-43.
ELWAZRI, A. M., P. WANJARA and S. YUE. The effect of microstructural characteristics of pearlite on the mechanical properties of hypereutectoid steel. Materials Science and Engineering: A. 2005, 404(1–2), 91-98.
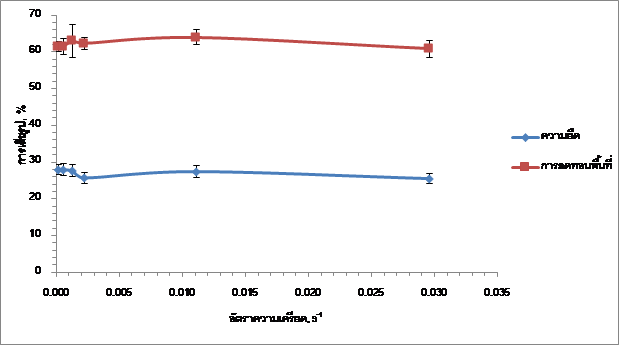
Downloads
Published
How to Cite
Issue
Section
License
Copyright (c) 2019 Department of Science Service

This work is licensed under a Creative Commons Attribution-NonCommercial-NoDerivatives 4.0 International License.